The sky is the limit —
As carbon capture gets cheaper, new applications could look attractive.
Scott K. Johnson
–
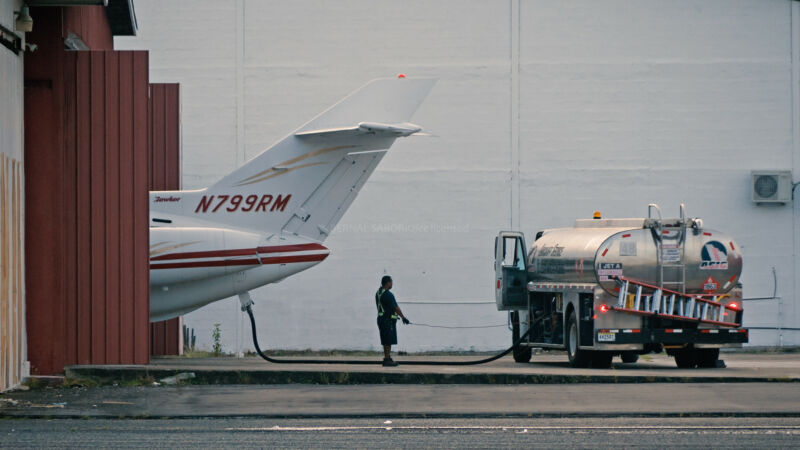
Techniques to take atmospheric CO2 and turn it into a fuel provide a climate-friendly alternative to exploiting fossil fuels—they may releases CO2 back into the air when burned, but there’s no net change. This includes biofuels crops, but can extend to industrial processes that directly involve CO2. As processes that capture CO2 from ambient air become more economical, so will the potential value of that CO2 as a resource for fuels.
There are a few ways to go about making fuel, but all require considerable energy because CO2 is a stable molecule—reversing the combustion reaction to make a new fuel doesn’t happen for free. But there’s an additional challenge: designing a process tuned to produce the exact type of fuel you want.
One way to do that is with a catalyst—a substance that guides the chemical reactions without being consumed by them. With the help of one catalyst, captured CO2 plus hydrogen gas might primarily be turned into methane; a different catalyst might shift the primary product towards the larger molecules of liquid fuels.
A new study led by Benzhen Yao at the University of Oxford describes a new catalyst that specializes in driving the production of the long-chain hydrocarbons used for jet fuel.
The numbers
That catalyst is an iron-manganese-potassium material—nothing too exotic. In tests, only five percent of the converted CO2 ended up as carbon monoxide and 10 percent ended up as methane, while nearly half turned into long-chain hydrocarbons in the jet fuel range (8-16 carbons). Compared to other catalysts tested in previous studies, that’s a much better output in the jet fuel range. And of the 2-4 carbon hydrocarbons produced, it favors alkenes over alkanes, which means a more carbon-carbon bonds and fewer carbon-hydrogen bonds. The reaction made five times as much propylene as propane, for example. Those are useful raw materials for things like plastics.
The catalyst provides sites to host reactions, influencing the product. Here, that’s primarily happening on an iron-carbon mineral that converts to and from an iron oxide. One form helps CO2 molecules react with hydrogen, while the other helps resulting carbon monoxide molecules react with hydrogen to build hydrocarbons.
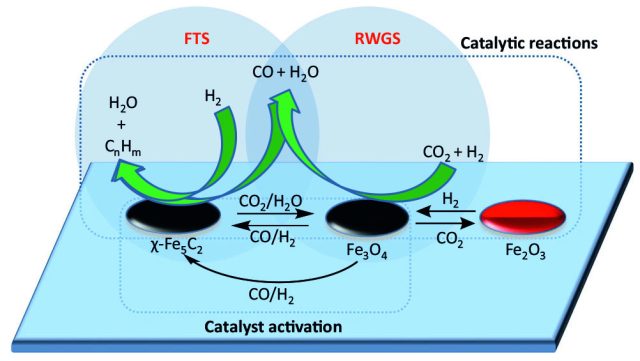
Enlarge / The basic chemistry at work here.
The method for making the catalyst turns out to be important. The materials were combined with an organic compound that is combusted while everything is kept at 350°C for a number of hours. The combustion reaction helps the iron, manganese, and potassium form the right minerals, and it leaves some carbon behind. Experiments with similar catalyst material made in a different way were far less effective.
A reset
Although the catalyst isn’t consumed during use, it does change, meaning it might have to be regenerated at some point. As before its first use, that can be done with high temperatures in the presence of hydrogen and carbon monoxide, resetting the mineral forms in the catalyst.
As part of a real-world system, this would be connected to a CO2 capture plant and an electrolysis device splitting water to make hydrogen gas. The products could then be separated and processed as needed.
Obviously, this study only relates to understanding what kind of catalyst to use, and there is no economic analysis of the fuel production operation here. There are a lot of energy inputs in this process, especially when compared to traditional oil production. But while petroleum-based fuels add to the CO2 in the atmosphere, air-based fuels could add very little (or none depending on where the required energy is coming from). And this also comes with the option of tailoring the types of hydrocarbons you make, rather than just working with whatever comes out of the ground.
Nature Communications, 2020. DOI: 10.1038/s41467-020-20214-z (About DOIs).